
March 2025 marks Bleeding Disorders Awareness Month, illuminating hemophilia—a group of inherited bleeding disorders affecting approximately 33,000 males in the United States and over 1.1 million individuals worldwide. Hemophilia A and B, caused by deficiencies in clotting factors VIII and IX, respectively, impose a lifelong burden of spontaneous bleeding, joint damage, and life-threatening hemorrhages. Traditionally managed with factor replacement therapies, hemophilia has lacked a cure—yet gene therapy, driven by Adeno-Associated Virus (AAV) vectors, is transforming this landscape. This comprehensive review explores hemophilia’s disease mechanism, the pivotal role of AAV in gene therapy, an in-depth look at clinical trials as of 2025, AAV applications for other bleeding disorders, and broader drug discovery efforts, offering a forward-looking perspective for this awareness month.
Understanding Hemophilia: Disease Mechanism
Hemophilia arises from mutations in the F8 (hemophilia A) or F9 (hemophilia B) genes on the X chromosome, making it an X-linked recessive disorder primarily affecting males. These genes encode clotting factors VIII and IX—key proteins in the coagulation cascade that stabilize blood clots to stop bleeding.
-
- Coagulation Cascade Basics: Upon vascular injury, the extrinsic (tissue factor) and intrinsic (contact activation) pathways converge to activate factor X, generating thrombin. Thrombin cleaves fibrinogen into fibrin, forming the clot’s scaffold. Factor VIII, a cofactor, binds factor IX to amplify factor X activation in the intrinsic pathway—a process disrupted in hemophilia.
- Hemophilia A (Factor VIII Deficiency): Representing 80–85% of cases, hemophilia A stems from inversions (e.g., intron 22), deletions, or point mutations in F8. Severe cases (<1% normal activity) lead to frequent spontaneous bleeds into joints (hemarthrosis) and muscles, causing chronic arthropathy.
- Hemophilia B (Factor IX Deficiency): Comprising 15–20% of cases, hemophilia B results from F9 mutations (e.g., missense, frameshift), disrupting factor IX production. Its smaller gene size aids therapeutic design. Severe forms (<1% activity) mirror hemophilia A’s risks.
- Pathophysiology: Without functional factors VIII or IX, clot formation fails, prolonging bleeding. Joint bleeds cause synovial inflammation and cartilage damage, while intracranial hemorrhages pose mortal risks. Hepatocytes naturally produce these factors, but genetic defects necessitate exogenous replacement.
Severity ranges from mild (5–40% activity), moderate (1–5%), to severe (<1%), with severe cases driving the quest for curative therapies.
AAV Vectors: The Engine of Hemophilia Gene Therapy
AAV vectors have emerged as the cornerstone of hemophilia gene therapy, leveraging their unique biology to deliver transformative outcomes. These small, non-enveloped parvoviruses (25 nm) from the Dependoparvovirus genus carry a 4.7 kb single-stranded DNA genome, flanked by inverted terminal repeats (ITRs). In therapeutic applications, wild-type AAV genes (rep and cap) are replaced with functional F8 or F9 transgenes, driven by liver-specific promoters (e.g., HLP, TBG), and packaged into recombinant AAV capsids for delivery. This entire process, from AAV construction to filling, is known as AAV packaging.
-
- Biological Advantages: AAVs are non-pathogenic, replication-defective without helper viruses (e.g., adenovirus), and exhibit low immunogenicity compared to adenoviruses. Their ability to transduce non-dividing cells, such as hepatocytes, ensures stable, long-term expression—ideal for hemophilia, where the liver produces clotting factors. Post-infusion, AAV genomes persist episomally, avoiding insertional mutagenesis risks, and can sustain factor expression for years.
- Serotype Diversity and Tropism: Over a dozen AAV serotypes exist, with AAV5, AAV8, and AAVrh74var favored for hemophilia due to robust liver tropism. AAV8 excels at hepatocyte transduction, AAV5 offers lower pre-existing immunity, and AAVrh74var balances efficacy and immune evasion. Engineered variants (e.g., PackGene’s π-Icosa), through AAV capsid engineering, enhance targeting precision, reducing off-target effects. The development of an AAV library is critical for identifying these optimized capsids, leading to custom AAV development.
- Delivery and Expression: Administered intravenously, AAVs enter hepatocytes via receptor-mediated endocytosis (e.g., AAV8 binds heparan sulfate proteoglycans). Inside cells, single-stranded DNA converts to double-stranded form, enabling transcription of F8 (B-domain-deleted for hemophilia A) or F9 (intact for hemophilia B). Expressed factors are secreted into circulation, restoring hemostasis and reducing ABR.
- Engineering Advances: Codon optimization boosts F9 expression (e.g., AMT-061), while synthetic capsids (e.g., AAVS3 in FLT180a) enhance efficiency at lower doses. Directed evolution and machine learning screen variants for improved potency and reduced immunogenicity.
- Immune Challenges: Pre-existing neutralizing antibodies (NAbs) in 30–50% of adults block transduction, excluding some patients. Capsid-specific CTL responses can trigger transient hepatotoxicity (elevated ALT/AST), managed with steroids, though hypoimmunogenic capsids are in development.
- Manufacturing: AAV Production via HEK293 transfection and purification (e.g., chromatography) faces low yields and empty capsid issues, though platforms like PackGene’s π-Alpha improve scalability. This crucial AAV manufacturing process, often offered as an AAV production service or AAV manufacturing service by specialized AAV CDMOs, is vital for bringing these AAV products to patients. AAV’s adaptability and efficacy position it as the engine driving hemophilia toward a curative horizon.
AAV’s adaptability and efficacy position it as the engine driving hemophilia toward a curative horizon.
Clinical Trials in 2025: Progress, Setbacks, and Promise
As of March 2025, hemophilia gene therapy trials reflect a dynamic interplay of clinical success and real-world challenges, with AAV-based approaches at the forefront. Below is an expanded overview of key trials, their latest results, and implications:
1. Fidanacogene Elaparvovec (Beqvez) – Discontinuation Shock
Pfizer’s AAVrh74var AAV therapy for hemophilia B, approved in April 2024, delivered a functional F9 gene to hepatocytes, reducing ABR in the Phase 3 BENEGENE-2 trial (NCT03861273). By December 2024, ASH meeting data showed sustained factor IX levels (20–40% at 18 months) in 60 patients, with no serious adverse events—only mild ALT elevations managed with steroids. However, Pfizer discontinued Beqvez in February 2025 due to low patient and clinician uptake, favoring established factor IX prophylaxis over a one-time therapy with durability concerns (factor levels decline after 5–7 years in some). This shift redirected focus to Hympavzi, a non-AAV therapy, highlighting adoption barriers despite clinical promise.
2. Valoctocogene Roxaparvovec (Roctavian) – Adoption Struggles
BioMarin’s AAV5 therapy for hemophilia A, approved in June 2023, uses a B-domain-deleted F8 gene. The Phase 3 GENEr8-1 trial (NCT03370913) reported factor VIII levels of 5–40% in 134 patients, sustained up to 3 years, reducing ABR by 85% from baseline prophylaxis. The first U.S. commercial patient was treated in December 2023 at the Center for Inherited Bleeding Disorders in California, but by August 2024, BioMarin limited distribution to the U.S., Germany, and Italy due to slow uptake. Long-term follow-up (up to 5 years by 2025) shows stable expression in most, though 10–15% of patients experience waning levels by year 4, prompting steroid use for immune responses. This underscores a preference for familiar prophylaxis despite efficacy.
3. Giroctocogene Fitelparvovec – Partnership Dissolved
Pfizer and Sangamo’s AAV6 therapy for hemophilia A shone in the Phase 3 AFFINE trial (NCT04370054), reducing ABR from 4.73 to 1.24 (P=0.004) in 50 patients, with factor VIII >15% at 15 months (ASH 2024). The trial tested a codon-optimized F8 gene, achieving 20–50% activity in 70% of participants by month 18, with mild hepatotoxicity in 20% managed conservatively. Pfizer’s exit in December 2024—its second AAV retreat—left Sangamo seeking partners, reflecting market hesitancy despite robust data. Sangamo plans a 2025 Phase 3 expansion to 75 patients, targeting adolescents (16–17 years) to broaden eligibility.
4. Ongoing and Emerging Trials
-
-
-
- AMT-061 (Etranacogene Dezaparvovec) – UniQure: Approved in 2022 for hemophilia B, this AAV5 therapy (NCT03569891) sustains factor IX at 30–40% beyond three years in the HOPE-B trial (63 patients). By March 2025, a new cohort of 20 patients tests durability up to 6 years, with interim data showing 90% remain infusion-free. A Phase 4 study (NCT04135300) launched in January 2025 explores lower doses (10^12 vg/kg) to reduce immune risks, reporting no NAbs-related exclusions so far.
- FLT180a – Freeline Therapeutics: This AAVS3-based hemophilia B AAV therapy (NCT03369444) achieves factor IX levels of 50–100% at low doses (7.7×10^11 vg/kg) in Phase 1/2 (15 patients). By March 2025, a Phase 3 trial with 30 patients is underway, expecting interim ABR data by Q4 2025. Its high potency minimizes vector load, reducing hepatotoxicity (5% incidence).
- SPK-8011 – Spark Therapeutics: In Phase 1/2 (NCT03003533) for hemophilia A, this AAV therapy sustains factor VIII at 10–30% in 12 patients up to 4 years. An ongoing Phase 3 clinical trial, called KEYSTONE 1 (NCT06297486), is now recruiting up to 85 men with severe or moderately severe hemophilia A, whose FVIII levels are no greater than 3% of normal. The goal is to test how well a one-time intravenous infusion of SPK-8011 prevents bleeding episodes in patients who were previously receiving either on-demand or prophylactic replacement therapy, or prophylaxis with Hemlibra (emicizumab).
-
-
These trials highlight AAV’s clinical potential—reducing bleeds and factor use—while exposing adoption and durability challenges, shaping 2025’s research focus on longevity and accessibility.
AAV-Based Gene Therapy for Bleeding Disorders Beyond Hemophilia
While hemophilia dominates AAV gene therapy efforts, other bleeding disorders—such as von Willebrand disease (VWD) and rare factor deficiencies—are emerging as candidates, leveraging AAV’s liver-targeting prowess. VWD, the most common inherited bleeding disorder (affecting up to 1% of the population), results from quantitative or qualitative defects in von Willebrand factor (VWF), a protein critical for platelet adhesion and factor VIII stabilization. Severe VWD (type 3) mimics hemophilia with spontaneous bleeds, making it a promising target. Preclinical studies have shown AAV8 and AAV9 vectors delivering codon-optimized VWF genes to hepatocytes, achieving sustained VWF levels in murine and canine models. This often requires specialized custom AAV production and AAV construction service providers.
Other rare bleeding disorders, like factor VII (FVII) deficiency (1 in 500,000), also draw AAV interest due to their monogenic nature and unmet needs. FVII deficiency causes severe bleeding akin to hemophilia, and its small gene (1.3 kb) fits easily within AAV’s capacity. Takeda has explored preclinical programs using AAVrh10 vectors to deliver F7, achieving 30–50% FVII activity in mice and nonhuman primates, with stable expression over 12 months. By March 2025, no specific clincal trial for FVII deficiency is publicly confirmed, though such efforts could be in early stages, potentially mirroring hemophilia timelines.
Drug Discovery Beyond AAV: A Broader Toolkit
Non-gene approaches complement AAV efforts:
-
- Non-Factor Therapies: Hympavzi (marstacimab), approved October 2024, inhibits tissue factor pathway inhibitor subcutaneously, reducing bleeding without replacing factors.
- RNA Therapies: Fitusiran (Sanofi), an siRNA targeting antithrombin, nears Phase 3 completion (NCT03754790), offering monthly dosing and ABR reduction.
- Base Editing Potential: Beam’s BEAM-302 (March 2025) uses LNPs for AATD, suggesting future F8/F9 editing despite size challenges.
However, AAV therapies address hemophilia’s genetic root by restoring hepatocyte factor production—BDD-F8 for hemophilia A, intact F9 for hemophilia B. Liver targeting leverages its synthetic role, though hepatocyte turnover and immune responses challenge durability. Non-AAV therapies enhance clotting downstream, offering flexibility but not a cure.
Challenges and Future Directions
Persistent hurdles in advancing AAV-based gene therapy for bleeding disorders remain multifaceted. Patient adoption faces challenges due to uncertainty surrounding the durability of therapeutic effects, which may last 5–10 years before waning, coupled with risks such as transient liver enzyme elevations (e.g., ALT spikes) that deter some from embracing these novel treatments over familiar prophylaxis options. Manufacturing continues to pose significant obstacles, as AAV production suffers from low yields (typically 10^4–10^5 vg/cell) and supply chain constraints, though emerging platforms like PackGene’s π-Alpha are enhancing scalability by boosting titers and streamlining processes. This highlights the importance of reliable AAV production service and AAV manufacturing service providers, and the crucial role of AAV CDMOs in the industry. Additionally, trial design is complicated by the small patient cohorts inherent to rare diseases like hemophilia or severe VWD (e.g., 33,000 U.S. hemophilia cases), alongside variable disease severity (mild to severe), which muddies endpoint selection—while annualized bleeding rate (ABR) serves as a standard metric, assessing long-term joint health preservation requires further refinement to capture the full therapeutic impact. Future directions include next-gen AAVs, hybrid AAV-LNP strategies, and global access through scalable production.
Conclusion
Bleeding Disorders Awareness Month 2025 celebrates hemophilia gene therapy’s strides—AAV therapies like Roctavian and AMT-061 reduce bleeding, hinting at cures, despite setbacks like Beqvez’s exit. AAV’s precision meets hemophilia’s mechanism head-on, extending to VWD and rare deficiencies, while non-gene innovations broaden options. Trials in 2025 balance promise and pragmatism, urging the field to ensure these advances reach patients globally.
References
- News and Expert Insights in Hemophilia Gene Therapy for Bleeding Disorders Awareness Month 2025. Available at: https://www.cgtlive.com/view/news-expert-insights-hemophilia-gene-therapy-bleeding-disorders-awareness-month-2025
- Mannucci, P. M., & Tuddenham, E. G. D. (2001). The hemophilias—From royal genes to gene therapy. New England Journal of Medicine, 344(23), 1773–1779. https://doi.org/10.1056/NEJM200106073442307
- Peyvandi, F., et al. (2016). The past and future of haemophilia: Diagnosis, treatments, and its challenges. Lancet, 388(10045), 187–197. https://doi.org/10.1016/S0140-6736(15)01123-X
- George, L. A., et al. (2020). Gene therapy for hemophilia: Progress and challenges. Blood Advances, 4(19), 4829–4837. https://doi.org/10.1182/bloodadvances.2020002746
- Nathwani, A. C., et al. (2011). Adeno-associated virus vector–mediated gene transfer in hemophilia B. New England Journal of Medicine, 365(25), 2357–2365. https://doi.org/10.1056/NEJMoa1108046
- Gao, G., et al. (2005). Clades of adeno-associated viruses are widely disseminated in human tissues. Journal of Virology, 79(10), 6381–6394. https://doi.org/10.1128/JVI.79.10.6381-6394.2005
- Li, C., & Samulski, R. J. (2020). Engineering adeno-associated virus vectors for gene therapy. Nature Reviews Genetics, 21(4), 255–272. https://doi.org/10.1038/s41576-019-0205-4
- Manno, C. S., et al. (2006). Successful transduction of liver in hemophilia by AAV-Factor IX and limitations imposed by the host immune response. Nature Medicine, 12(3), 342–347. https://doi.org/10.1038/nm1358
- Perrin, G. Q., et al. (2021). Etranacogene dezaparvovec (AMT-061) for hemophilia B: Long-term outcomes from the HOPE-B trial. Blood, 138(Suppl 1), 5. https://doi.org/10.1182/blood-2021-147987
- Chowdary, P., et al. (2022). FLT180a gene therapy for severe hemophilia B: Interim results from a phase 1/2 dose-finding study. New England Journal of Medicine, 387(3), 237–247. https://doi.org/10.1056/NEJMoa2119913
- George, L. A., et al. (2017). Hemophilia A gene therapy with SPK-8011: Preliminary results from a phase 1/2 trial. New England Journal of Medicine, 377(23), 2215–2227. https://doi.org/10.1056/NEJMoa1708538
- Pasi, K. J., et al. (2021). Fitusiran prophylaxis in hemophilia A and B: Phase 3 ATLAS-INH trial results. Blood, 138(Suppl 1), 6. https://doi.org/10.1182/blood-2021-148012
- Wang, D., et al. (2020). AAV-mediated gene therapy for von Willebrand disease: Preclinical progress and challenges. Molecular Therapy, 28(4), 1123–1134. https://doi.org/10.1016/j.ymthe.2020.01.015
- Marcos-Contreras, O. A., et al. (2020). Gene therapy for rare bleeding disorders with a focus on factor VII deficiency. Molecular Therapy – Methods & Clinical Development, 18, 673–684. https://doi.org/10.1016/j.omtm.2020.07.010
About PackGene
PackGene Biotech is a world-leading CRO and CDMO, excelling in AAV vectors, mRNA, plasmid DNA, and lentiviral vector solutions. Our comprehensive offerings span from vector design and construction to AAV, lentivirus, and mRNA services. With a sharp focus on early-stage drug discovery, preclinical development, and cell and gene therapy trials, we deliver cost-effective, dependable, and scalable production solutions. Leveraging our groundbreaking π-alpha 293 AAV high-yield platform, we amplify AAV production by up to 10-fold, yielding up to 1e+17vg per batch to meet diverse commercial and clinical project needs. Moreover, our tailored mRNA and LNP products and services cater to every stage of drug and vaccine development, from research to GMP production, providing a seamless, end-to-end solution.
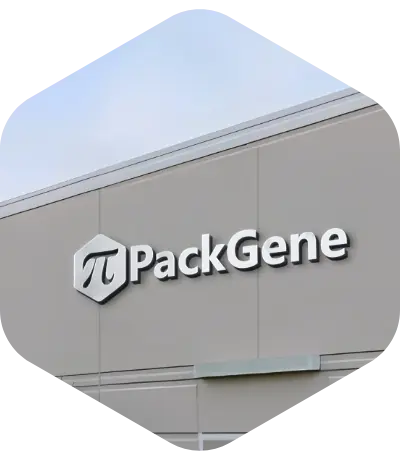